In the beginning…
We observed and harnessed electricity way before we understood how it worked. Electricity is a range of phenomena associated with the flow of charge.
See the critical thinking activity, Activity 1, What is electricity, that gets students to think critically about how electricity has changed the world, where it comes from and how we generate and use electricity.
To understand what electricity is and how it works, it helps to understand the structure of the atom, knowledge that the scientists of the 17th through to the 19th centuries lacked when developing their ideas about the nature of electricity.
Get students to draw an atom that will help teachers establish students’ baseline understanding of the structure of the atom. Activity 2, the critical thinking and Draw an atom activity is here.
After drawing an atom, take students on an in-depth examination of the atom and the scientific journey to understand its structure, a journey that reveals how scientists got it wrong a lot of times before reaching the understanding we have today. And we are still working out the finer details.
The atom and the electron
Atoms have a core (nucleus) that is made up of protons and neutrons. The protons carry a charge, the neutrons are neutral – no charge. Surrounding the nucleus is a cloud of charged particles known as electrons. Electrons have charge that is opposite to the charge on the proton.
It was Benjamin Franklin in 1748 that suggested there were two types of charge that were opposites*. The opposite charges attracted each other and like charges repelled each other. We now call these charges, positive and negative, but we could easily call them Bob and Jenny or Ping and Pong. At the time, Franklin had no means to measure or quantify his ideas, but it turned out he was correct – sort of. What Franklin got wrong was his suggestion that current flowed from the positive to the negative. And Franklin and his contemporaries at the time used the concept of flow in the literal sense because electricity was considered a form of invisible ethereal fluid. It was not until 1897 when J. J. Thompson experimentally determined the existence of the electron using devices called cathode ray tubes that we realized Franklin’s mistake. See the section, ‘Finding the electron’ below. Soon after finding the electron we learned that it was the loss and gain of electrons from the atom and the movement of the electron being drawn toward a positive charge that generates the flow of charge (electricity) in a circuit. But the convention of understanding that charge travels from the positive to the negative has stuck and we now have two conventions: the conventional model (charge flows from positive to negative) and the electron model (charge flows from negative to positive). It can be confusing, but the important point is that the math is the same and the outcome is the same whichever model is applied. This unit will apply the electron model in its explanations and activities.
*While probably the more famous and the one credited with the idea of charged particles, there were other natural philosophers before Benjamin Franklin that used language to describe phenomenon similar to Franklin’s ideas on charges and electrification. A slightly more academic review article that gives one historical perspective and overview on the history of electricity is Marton and Marton, Evolution of the Concept of the Elementary Charge. Editor(s): L. Marton, C. Marton, Advances in Electronics and Electron Physics, Academic Press, Volume 50, 1980, Pages 449-472.
Understanding the atom
Proton says to the electron: “I don’t feel well.”
Electron: “Are you sure?”
Proton: “I’m positive.”
Our understanding of the atom today went through a few iterations over a few decades. J.J. Thomson was the first to experimentally determine the existence of the electron, but his model of the atom was still based on limited data and understanding, and consequently wrong. In his model, he had the charged particles (electron and protons) all mixed in together in something akin to a charged soup, or as it was dubbed, a “plum pudding” See Figure 1. Later we learned that an atom was mostly empty space, which is the knowledge that led us to our current understanding of the atom that has a nucleus where the protons and neutrons sit. Buzzing randomly around the nucleus in discrete energy levels are the electrons, with lots of space between the electrons and the nucleus.
Imagine a tennis ball in the centre of a football ground. Inside the hollow tennis ball are all the protons and neutrons. Out in the grandstand where the crowd sit is the cloud of electrons randomly buzzing around the outside of the ground. This is a rough approximation of the distance between the nucleus and electrons and why atoms and therefore everything is mostly empty space. For the older or more advanced students you can talk about each grandstand tier representing a distinct energy level or shell that the electrons are confined to as they move randomly though the tier that corresponds to their given energy level. The number of protons an atom has will determine what element it is. For example, Hydrogen has one proton, Helium has two, Lithium has three…and so on. The atom is most stable when it has the same number of protons and electrons that give it a neutral charge. Some atoms will lose or donate an electron and become positive. Others can gain an electron and become negative. Charged atoms are called ions. Oppositely charged ions can form bonds with each other. For example, Na+ (sodium) and Cl- (Chloride) when bonded make salt (NaCl).
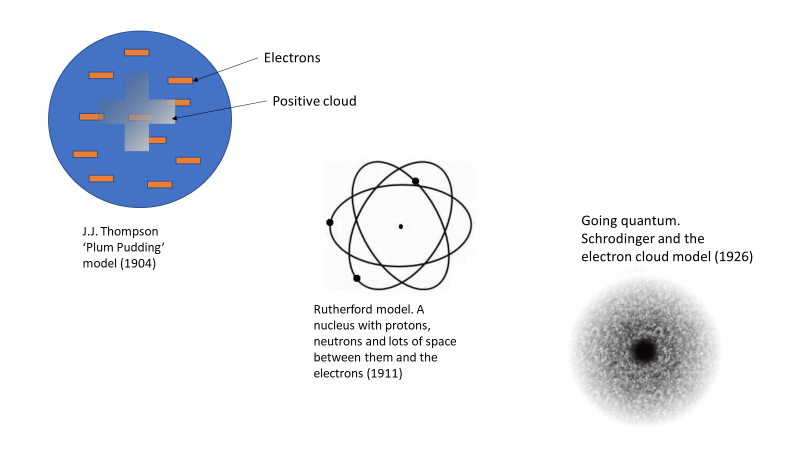
Figure 1. Atomic models starting from J.J. Thompson’s plum Pudding in 1904 through to Schrodinger’s electron cloud in 1926.
There are loads of images on the web of the different atomic models as they were developed over time. Here is one
Try the activity, Build an atom, for more detail about the nature of charge and an opportunity to make an edible atom model. The concept of charge will also be elaborated on as we move through the activities. Students will learn more about some of the scientists involved in making the initial discoveries that led to our current understanding of electricity.
The quantum model of the atom
The electron cloud model of the atom attempts to represent the quantum nature of the atom – always a tough ask because we have to get our heads around the concept of superposition.
For our purposes we will focus on the electron. Many definitions of superposition state it as a system being in multiple states at the same time. But something can’t really be in multiple states, such as position, at once. It is all about probability. We have really good and well understood math that enables us to calculate the probability of the position and momentum of an electron (or quantum state). We can measure and calculate the probability that an electron will have a certain state (eg, position or momentum). This is very different to it being in or having multiple states at the same time.
The electron also has a wave function. That it, it behaves as a wave rather than a particle. As a wave it still has the quantum version of a position, momentum and energy level.
Quick activity – a role play
Get students to examine a Rutherford model of the atom – which is the one they will likely be most familiar with (and the one they will typically draw if you ask them to draw an atom).
Ask students where on the Rutherford model the electrons are. Students will inevitably point to a dot/particle in the image that represents an electron.
Role play. Select an element from the periodic table (keep it simple and select H, He or Li).
Get students to play the neutrons and protons in a nucleus. In helium, for instance, this will require 2 student protons and 2 student neutrons. They will need to stand close together to simulate a nucleus. Technically if you simulated a neutral atom, you will need two electrons. But for this exercise, you will just simulate one. You will need about 7-10 students to represent this one electron. Get the students to form a circle around the nucleus and join hands. Get the students representing the electron to do the Mexican wave. Remember the student electrons doing the wave represent just one electron. As a (Mexican) wave they surround the nucleus. Ask the remaining students to now point to the electron.
This should get the students thinking because they cannot definitively say the electron is in a particular point in space like they can in the Rutherford model.
Check out Minute Physics modelling of the quantum atom below – a thing of beauty.
And before you explore in more detail the nature of electricity, get students to consider what they think electricity is. See Activity 4, What is electricity. Draw a circuit that establishes a baseline understanding of circuits and electricity. It can be compared to their understanding at the end of the selected activities where students get to build a real circuit. Now we go back further in time to examine how we first perceived the concept of electricity.
How we built an understanding of static electricity
Apart from our observation of lightning, the ancient Greeks were probably the first to at least record their experience with static electricity that they themselves generated by rubbing amber with a cloth. As noted below, we did not at the time understand that lightning was electricity and that it and the sparks coming from the amber were one and the same thing. That understanding came much later.
Francis Hauksbee, a scientific instrument maker in the early 18th Century was one of the first to build a machine to demonstrate the concept of static electricity. He built a machine that rotated a hollow glass sphere that had the air sucked out by an air pump (also designed by Hauksbee). When you placed your hands on the rotating glass sphere, the machine generated static electricity that could be seen as an aura within the sphere. It was demonstrated to an audience of the Royal Society and it was one of the first demonstrations to suggest that electricity could be something other than amusement, though the initial use of Haukebee’s machine was by entertainers who used electricity to perform what we would consider magic shows.
Back then people who experimented with electricity or used it to make entertainment called themselves electricians.
Stephen Gray has been called ‘the father of electricity’. In the 1720s and 30s, he used one of Hauksbee’s rotating spheres to demonstrate that he could create a charge and transfer that charge to another object and that electricity could be conducted through some materials, but not others. For a deeper insight check this Royal Society blog.
It was not long after this that Benjamin Franklin proposed an experiment that the French actually carried out, which demonstrated that lightning was actually electricity, the same stuff that Gray, Hauksbee and the ancient Greeks had made. They erected a long metal rod in a frame, one end pointing the sky, the other end insulated from the ground in the frame. Then they waited for a storm. One came and lightning struck the metal rod, storing some of that electrical energy in the rod. Just after the lightning struck the rod, one brave French person ran out and put their hand close to the rod and electricity jumped across the rod to their hand, giving the poor unfortunate and shock and minor burn, but ultimately showing that lightning was indeed electricity. It was these experiments and observations that validated Ben Franklin’s assumption and eventual development of the lightning rod that we now have on all buildings to protect them from lightning.
We still did not know how electricity worked. We were still only observing the phenomenon.
What we know now is that static electricity, or any electricity is the movement of charge (usually the electron) through a material. With static electricity, electrons build up on an object or area until there is sufficient potential or attractive force for a charge (electrons) to jump to an area with an overall positive charge. This is visualized in the form of a spark. In the case of static electricity that movement of electrons is through air and air is a good insulator, which is why in the case of lightning you needs loads of electrons to build up in the clouds before they can make the explosive jump (a really big spark) all the way to the ground that is acting as the positively charged object (or less negative than the electron charged atmosphere of the sky).
It would be another century before we started to understand how electricity worked and that it was intimately connected to magnetism. See the section below on electricity and magnetism.
See Activities 5 and 6, Static, sticky and flappy plastic, and Water Bender to witness static electricity in action and help students conceptualize charge and the movement of charge.
Conductors, insulators and resistance
We have noted that how tightly bound electrons are to their atom influences how good they are at conducting electricity. This mobility is based on how easily electrons that are in the outermost valence shell – the electrons furthest from the nucleus – can leave their atom and move through the material.
An insulator is a material whose atoms have their electrons tightly bound to the nucleus and are unable to easily free themselves to flow through the material to generate electricity.
A conductor is a material that has electrons in the outer shell that are loosely bound to their atoms which enables the electrons to flow easily through a material when an electrical force (voltage) is applied. Metals are good conductors for this reason. But even good conductors have some level of what is known as resistance that will impede the flow of electrons through the material.
Resistance
Resistance is a property of the material that impedes the flow of electrons. Therefore, the greater the resistance of a material, the more energy is required to get electrons to flow through that material and fewer electrons will be moving. There will be a reduced flow of charge. See Figure 2. Where there is resistance – and there is always some – energy in the electron is lost as heat rather than being used to perform the task we want such as powering the TV or computer. The heat is generated as electrons flow through a material and interact with and transfer their kinetic energy to atoms in the atomic lattice. The energy gained by the atoms in the lattice makes them jiggle about a lot more and this kinetic energy is transformed into heat. Materials with high resistance can make good insulators because of the difficulty to generate any flow of charge. Materials with low resistance make good conductors because it requires only low voltages, or energy, to get the electrons moving and generate electricity.
Quantum look at resistance
As noted, even the best conductors in today’s circuits have some resistance. You can feel this resistance as heat.
Phonons and resistance
Electron waves interact with the atomic lattice waves (phonons) and transfer some of their energy and momentum into the lattice waves. Phonons are similar to a sound wave that an atom will give off as it vibrates, which all atomic lattices and atoms do unless they are cooled to absolute zero, which is theoretically impossible. This extra energy gained by the atomic lattice makes the lattice jiggle a bit more and this greater kinetic energy = loss of energy as heat. The electrons have lost energy in the process, which slows their flow through a circuit and therefore generates less electrical energy.
In room temperature metals/conductors, nearly all the resistance is from phonons. See Figure 3 below.
*It is important to note to students that electrical energy is not the flow of electrons (current). The kinetic energy of electrons moving through a circuit is what enables the generation of the electrical energy used to do the work. To understand more about the concept of energy and work see the section, Energy and work in the FLEET Schools resource on Energy
Ask students what they feel when they use their mobile or laptop for a while? What are the devices giving off? Hopefully they eventually say, heat. This heat is resistance and lost energy. It is energy unavailable to do work.
See Activity 7 Conduct and resist; the dance. There are two main objectives with this activity: to help students visualize how resistance works at the quantum level, and to challenge potential student understanding of resistance, which is typically based on the classic model of the atom and the intuitive concept of the electron as a particle.
What would the implications be if we could conduct electricity without resistance?
In Activity 8, Life without resistance, students have to think critically about what it would mean if we could conduct electricity without resistance.
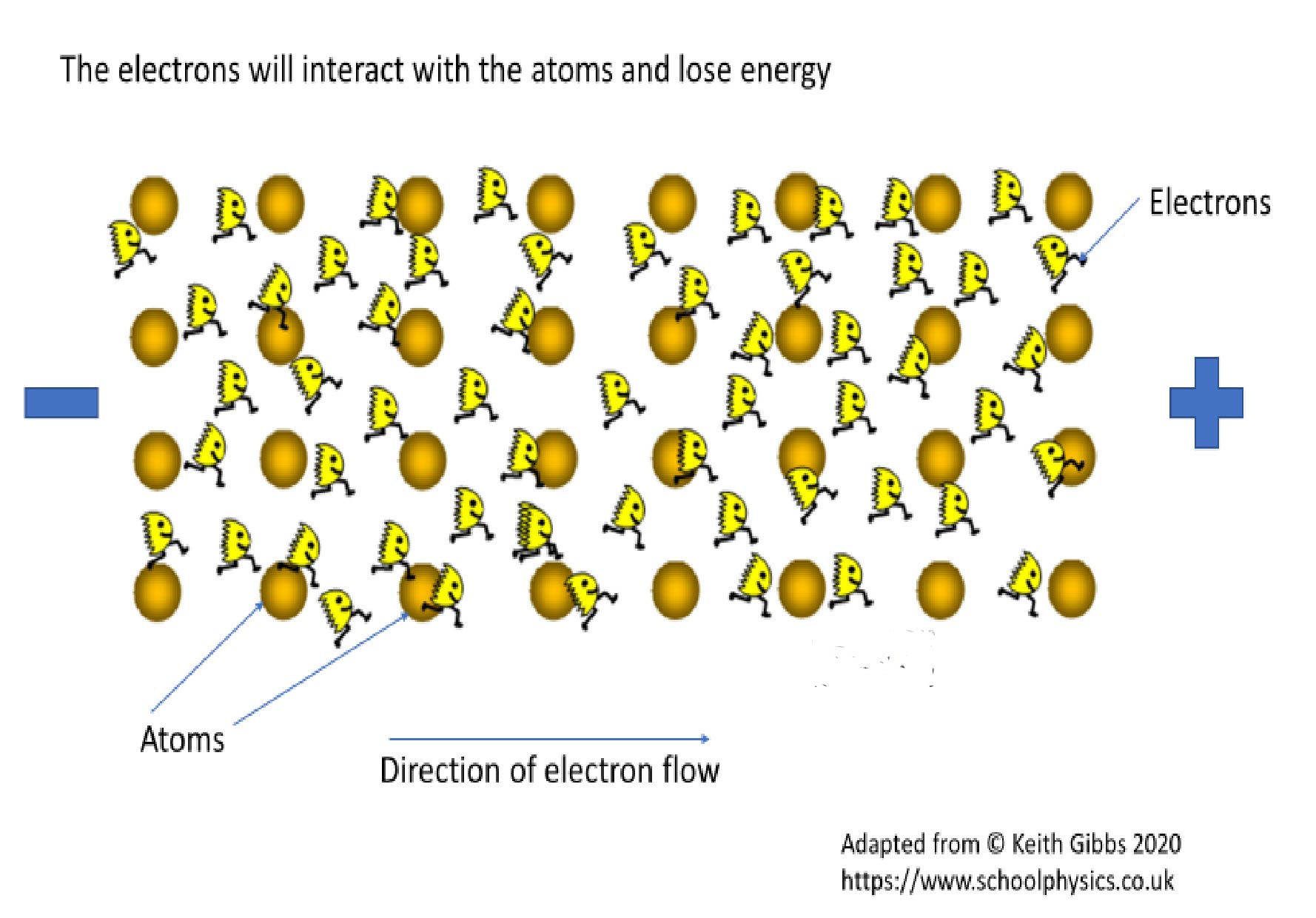
Figure 2. The greater the interaction of electrons with other atoms and the tighter the electrons are bound to their atom, the greater the resistance.
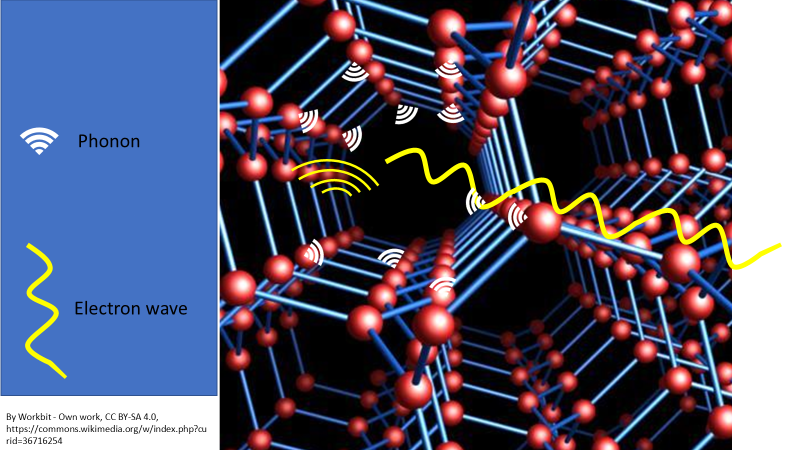
Figure 3. Electrons have a wave-like behaviour. They don’t actually physically hit the atoms as they flow through a circuit. The electron wave interacts with the phonon (which is a form of sound wave) that is given off by a vibrating atom. Energy is passed from the electron to the atom via these waves
Resistance is behind what we call “compensatory emissions.” These emissions are the result of the extra electricity – often generated from fossil fuels – required to compensate for energy lost throughout out grid because of resistance. It has been calculated that worldwide, compensatory emissions amount to nearly a billion metric tons of carbon dioxide equivalents a year, in the same range as the annual emissions from heavy trucks or the entire chemical industry.
Energy loss through transmission alone ranges from 2-50% depending on the country. [link]
In Australia, energy lost through transmission is about 10%. That figure would be greater the further you are from the source. [link ]
Bringing in the next tech generation – zero resistance
There are some materials that when cooled down to really cold temperature (about -170 degrees Celsius) will conduct electricity with zero resistance. These materials are called superconductors. The fact they only exhibit this behaviour at such low temperatures makes them impractical for most purposes, but the goal of researchers in this field is to develop materials that can superconduct at room temperature.
The 2D materials and superfluids that FLEET is working on will conduct with near-zero resistance at room temperature and are alternatives to the superconductor. But we are only just beginning to learn about the potential of these materials and there is still a lot of research to go before we can realize that potential and use such materials to develop energy-efficient electronics. See FLEET research and the need for the next generation of electronics.
Head to the next section in the unit, Electricity and magnetism
Back to FLEET Schools